In this article we explore the current use of hydrogen in industrial processes, as well as its role in the ongoing global transition to a net-zero economy. We’ll provide insights into market opportunities and challenges, the latest processing technologies, and best practices in safety to help industry stakeholders as they face mounting pressure to scale up hydrogen production.
Hydrogen can be very dangerous. At every point across its value chain, upstream and downstream, hydrogen remains extremely combustible and can explode in confined spaces when exposed to any electrical equipment producing arcs, sparks, or excessive heat. Consequently, correctly certified explosion-proof electrical equipment is required as defined in the NFPA 70 National Electrical Code (NEC Article 500), among others, depending on geography. For the purposes of this article, we will concentrate on NEC requirements for hydrogen, specifically Class I, Division 1 or 2, Group B.
The Hydrogen Market
According to the International Energy Agency, hydrogen represents “the biggest innovation opportunity” to reduce or eliminate carbon dioxide (CO2) emissions produced by human activity. Hydrogen’s ability to replace fossil fuels without releasing CO2 when consumed has led to new applications being explored on numerous fronts, notably fuel cell electric vehicles. Bolstering these initiatives, the U.S. Department of Energy (DOE) in 2023 announced an investment of $7 billion to launch seven Regional Clean Hydrogen Hubs to accelerate the commercial-scale deployment of low-cost, clean hydrogen.
While green innovations like these hold great promise, hydrogen as an alternative energy represents only a small sliver of overall consumption. Of the 120 million metric tons (MMT) of hydrogen consumed worldwide in 2022, the vast majority was concentrated in the traditional sectors of petroleum refining and chemical processing.
Globally, each year approximately 55% of hydrogen goes into the creation of ammonia for fertilizer, another 25% into petroleum refining hydro-desulfurization and hydrocracking operations, 10% to produce methanol, and the remaining 10% in treating metals, flat glass manufacturing, chemical synthesis of plastics, and the manufacturing of semiconductors. Gaseous hydrogen is also utilized as a coolant for generators due to its high thermal conductivity. Almost all the hydrogen produced in the U.S. is used for refining petroleum, treating metals, producing fertilizer, and processing foods.
Hydrogen Production
Hydrogen is the simplest and lightest of all Earth elements, consisting of only one proton and one electron. It is also the most abundant element in the universe, yet rarely in its pure state. Instead, hydrogen covalently bonds to one of three elements: fluorine, oxygen, or nitrogen. To produce hydrogen as fuel, it must be separated from the other elements in the molecules where it occurs. Technologies enable the separating of hydrogen gas from its companion substances in purities in the order of 99.999%.
Hydrogen is almost exclusively produced through thermochemical processes requiring fossil fuels: hydrocarbon reforming, coal gasification, hydrocarbon pyrolysis, and plasma reforming. Approximately 95% of hydrogen is produced via steam reforming of hydrocarbons (Figure 1) with the balance coming from water electrolysis, biomass-derived liquid reforming, and microbial biomass conversion.
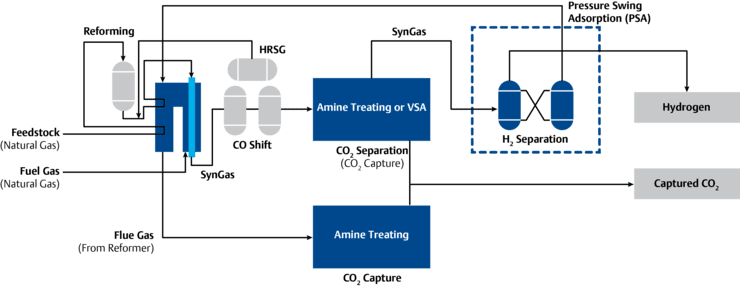
The least expensive method of production is steam methane reforming (SMR). Besides methane, it is used to produce hydrogen from ethanol, propane, and gasoline. Although steam refining is very cost-effective, it requires fossil fuels as feedstock and to produce heat. In fact, for every kilogram of hydrogen produced in SMR, seven kilograms of CO2 are released into the environment. Carbon capture and storage (CCS) techniques seek to trap the vast majority of CO2 produced by SMR and store it in the ground, converting the product from “gray” to “blue” hydrogen (see sidebar).
Hydrogen Production Color Codes
Different methods of producing hydrogen are often referred to by certain colors:
|
An alternative to steam refining is water electrolysis. This pathway splits hydrogen from water using electrical current, effectively converting electrical energy into chemical energy. Depending on the kind of electrolyte material and ionic species being used, electrolysis is carried out using one of three types of electrolyzers: alkaline, solid oxide, or proton exchange membrane (PEM).
Like steam refining, electrolysis largely relies on fossil fuels. The only way the process is CO2 neutral—that is, it produces almost no greenhouse gases or other pollutants—is when renewable energy sources are employed, such as wind or solar. Because this “green” hydrogen approach is considerably more expensive than hydrogen sourced from fossil fuels, it makes up less than 0.04% of all hydrogen produced globally.
Hydrogen Storage
Hydrogen may be physically stored as a gas (Figure 2) or a liquid, yet both methods present challenges. Low energy density and the complexities of cryogenics are some of the biggest barriers. Hydrogen gas is prone to leakage. It can permeate through solid metals, as well as embrittle metals, which affects the integrity of storage and transportation infrastructure by creating cracks. Even a tiny leak can lead to the accumulation of dangerous hydrogen clouds in a confined area or within an enclosure.
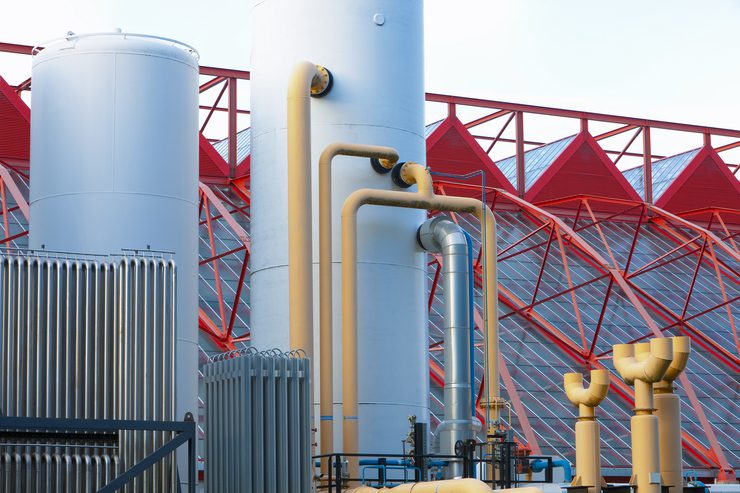
Another storage option is to chemically bind hydrogen with solids or liquids able to absorb it. Finally, there is the underground storage of hydrogen. Bulk storage of uncompressed hydrogen has proven viable in salt caverns, and to a lesser extent in porous media such as sandstone or shale, and in engineered cavities.
Hydrogen Transportation
Most hydrogen used in the U.S. is produced at or close to where it is consumed—typically at petrochemical or chemical sites. If it isn’t consumed at its point-of-production, hydrogen can be transported to its point-of-use by railcar, barge, or over the road in gaseous tube trailers or in cryogenic liquid tanker trucks.
Long-distance pipeline investments have been made in areas where there is a significant, steady demand for hydrogen. In the U.S., there are approximately 1,600 miles of active pipeline for hydrogen transportation, 90% of which is located along the Gulf Coast of Texas, Louisiana, and Alabama, serving refiners and ammonia plants. Pipelines are the least expensive method of transporting hydrogen.
Hydrogen Fires and Explosions
When developing electrical systems for a hydrogen application, engineers must be aware of its associated dangers and the need for proper hazardous area classification for electrical equipment. Make no mistake—there are serious safety concerns when hydrogen is released in sufficient concentrations, a situation made more precarious because hydrogen is both colorless and odorless.
Hydrogen’s wide flammability range (4% to 74%) means the energy needed to ignite it can be very low, like that generated by a small spark or an electrostatic discharge. Therefore, all electrical equipment must be bonded and grounded. Any equipment that is not approved for hazardous environments by the NEC, IECEx or ATEX must be kept outside of the designated area.
Another danger of hydrogen is that its flames burn at extremely high temperatures (500C, 932F), yet, mostly outside of the visible light spectrum, making these fires both extremely destructive and nearly impossible to initially detect. Furthermore, at concentrations of 18.3% to 59%, hydrogen will explode. Blast waves from a hydrogen explosion can cause very serious damage to surrounding buildings as well as injuries to nearby personnel.
Explosive forces will vary depending on several factors, such as the quantity of gas, the presence of any other materials, and the conditions under which the explosion occurs including container geometry. The DOE Office of Energy Efficiency and Renewable Energy classifies hydrogen explosions as being either deflagrations or detonations (see sidebar).
Types of Hydrogen Combustion: Deflagration Versus Detonation
The following compare and contrast deflagrations and detonations:
|
Hydrogen has one safety advantage over other flammable fuels: hydrogen is 14 times lighter than air and rises six times faster than natural gas, which means that it disperses rapidly when released. Unless leaking hydrogen is contained by a roof or some other structure, it will quickly disburse before it reaches a flammable concentration. The laws of physics prevent it from lingering near a leak unlike heavier gases such as propane or gasoline fumes. For that reason, hydrogen explosions and fires are most likely to occur in confined, poorly ventilated spaces where hydrogen is processed or stored in large quantities.
Mitigating Hydrogen Risks
In North America, electrical systems installed in hydrogen applications follow NEC Article 500, as previously mentioned. The NEC is incorporated into most state and local jurisdiction regulations and therefore carries the weight of law.
In Article 500, 502, and 503, the NEC details its Class (nature of the hazardous material) and Division (likelihood of existence of it) system, which is further delineated into Groups (the type of hazardous materials). The NEC Class/Division System for hazardous areas classifies hydrogen applications as being Class I, Division 1 or Division 2, Group B (Figure 3).
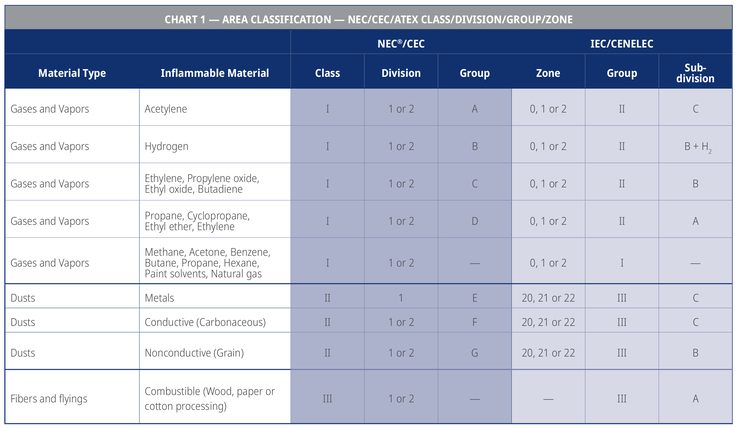
A Class I area is defined by the NEC as, “Locations which are deemed hazardous due to the presence of gases or vapors that are present in the air in a sufficient quantity to produce explosive or ignitable mixtures.” This will apply to most areas where hydrogen is produced, stored, transported, or dispensed.
Class I hazardous locations are subdivided into Divisions. Division 1 is a Class I location “where the hazardous atmosphere is expected to be present during normal operations on a continuous, intermittent, or periodic basis.” Division 2 is a Class I location in which “volatile flammable liquids or gases are handled, processed, or used but in which they would normally be confined within closed containers or closed systems from which they can escape only in the event of an accidental rupture or breakdown of the containers or systems.”
Divisions are broken down into seven groups (A, B, C, D, E, F, and G), although three (E, F, and G) refer only to dust and not gases. Data pertaining to combustible vapors and gases in determining Group are its flash point, upper/lower flammable limits, auto-ignite temperature, density, and pressure, as well as its MIE (minimum ignition energy), MIC (minimum igniting current), and MESG (maximum experimental safe gap).
Hydrogen falls into Group B. Group B is an area that contains a flammable gas, liquid-produced vapor, or combustible liquid-produced vapor mixed with air that has the potential to catch fire and burn or explode. The elements in this Group have an MESG value equal to or less than 0.45 millimeters or an MIC ratio equal to or less than 0.40. Besides hydrogen, other Group B gases include butadiene, ethylene oxide, propylene oxide, and acrolein.
Applying due diligence in the classification, design, and installation per the applicable code will render hydrogen no more dangerous than any other product. We’ll also note here that the application of ventilation may change the electrical requirements in hazardous locations where hydrogen is present, as can walls, ceilings, and floors as separation between classified and non-classified areas. If fans are being used, vent fan blades should be constructed with aluminum, plastic, or other non-sparking material.
Hazardous Area Strategy
The following safety guidance is recommended:
|
Explosion-proof Equipment Design
Explosion-proof equipment for Class I, Division 1 or 2, Group B applications are designed and manufactured strong enough to contain an explosion and prevent the escape of a flame or heat that could ignite the surrounding atmosphere. Burned gases do escape from explosion-proof equipment. However, their escape path has been engineered so the temperature of the escaping gas is well below its ignition point when it escapes into the surrounding atmosphere. This can be done in several engineering methods depending on the product, such as labyrinth-path and ground joint construction.
Other means of ensuring equipment is explosion-proof are sealing it to prevent entry of flammable gas, encapsulation in resin or oil immersion, pressurization with inert gas to displace hazardous substances, or limiting the temperature of its external surfaces. Electrical equipment subjected to this environment must also provide protection from the ingress of liquids or dust to maintain proper operation.
Reducing the risk of hydrogen explosions and fires requires classifying areas of the operation as hazardous. Because hazardous area classification can be a complex and daunting task, consult NFPA 70 (National Electric Code), NEC Articles 500–516, and NFPA 497 “Recommended Practice for the Classification of Flammable Liquids, Gases, or Vapors and of Hazardous (Classified) Locations for Electrical Installations in Chemical Process Areas.”
—Bogdan Moldovan is product marketing manager, Emerson Automation, Appleton.
- SEO Powered Content & PR Distribution. Get Amplified Today.
- PlatoData.Network Vertical Generative Ai. Empower Yourself. Access Here.
- PlatoAiStream. Web3 Intelligence. Knowledge Amplified. Access Here.
- PlatoESG. Carbon, CleanTech, Energy, Environment, Solar, Waste Management. Access Here.
- PlatoHealth. Biotech and Clinical Trials Intelligence. Access Here.
- Source: https://www.powermag.com/ensuring-electrical-safety-in-hydrogen-operations/